The magical three-dimensional (3D) printing technology in science fiction movies such as Star Trek, Jurassic Park III, and Westworld has now become reality — 3D printed cars, 3D printed skulls, 3D printed hearts, and 3D printed prosthetics are just some examples. The first multi-material 3D printer for printing food materials such as chocolates, cookie doughs, and cheeses was created at Cornell University in 2006 (Liu et al., 2017). Since then, the use of 3D food printing has been growing rapidly not only in laboratories but also in commercial sectors due to reduced costs and further developments in technology.
What is 3D food printing?
3D printing, also known as additive manufacturing, is the process of constructing a 3D object by using a computer-aided-design (CAD) model or a digital 3D model. This is a process which involves the addition of raw materials, deposition, and solidification, typically layer-by-layer under the computer’s control (Dankar et al., 2018).
In 3D food printing, food ingredients are raw materials, and a printer typically includes three parts: a printing system, an operation control platform, and a food capsule (Baiano, 2020). The food capsule holds the food material, usually a thick slurry analogous to printer ink, that is forced through a food-grade nozzle by a syringe pump to deposit on a platform, layer-by-layer, according to a pre-designed shape controlled by a computer (Figure 1). The nature of the food ingredients and sanitary status of the printing device determine if the printed food can be consumed directly or needs additional cooking (Baiano, 2020).
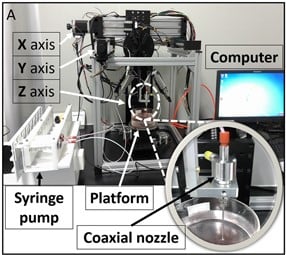
Figure 1: An example of a 3D bioprinting system containing a three-axis platform, a syringe pump, a coaxial nozzle, and a computer controller (Dai et al., 2017)
The ability of consumers to personalize what they eat and how their foods look is an exemplary advantage of 3D food printing, while some current disadvantages include the cost, availability of “food inks”, and safety concerns. Further considerations are listed in Table 1, below.
Table 1: Some advantages and limitations of current 3D food printing technology (Pereira et al., 2018)Advantages | Disadvantages |
|
|
This article was written by Shuang Ren, PhD student, and Qixin Zhong, PhD and Professor, both at the Department of Food Science, The University of Tennessee, USA.
What kinds of food can be “printed”?
So far, more than 30 food products have been successfully printed, including confectionery such as chocolates and fudge, baked goods such as biscuits and cakes (Figure 2), snacks such as potato chips, fruit and vegetable products including various purees, juices, as well as jellies, meat products and dairy products such as cheeses and yoghurt (Dankar et al., 2018).
Figure 2: Examples of 3D-printed chocolates and cookies (source: http://www.shiyintech.com/site/scene)
Technologically, the flow and deformation properties, i.e., rheological properties, of the “food ink” are one of the most crucial factors in 3D food printing (Figure 3). To be pumpable and extruded from the nozzle, the ink must be thin enough; whereas, after depositing on a surface, the ink must provide mechanical support in order to maintain the shape of the printed product (Chen et al., 2022).
Figure 3: Rheological properties impacting the printability of food materials (Chen et al., 2022)
In rheology, inks with such properties are called Bingham plastic or Herschel-Bulkley materials. At rest, these materials form a weak network to self-support their weight to maintain the shape. Once the network is disrupted by a sufficiently high stress (force acted on a unit area; generated by the syringe pump), known as yield stress in rheology, the ink has a low enough viscosity to enable printing (Chen et al., 2022).
When measured with a rheometer, most printable materials show shear thinning properties, i.e. a lower viscosity at a faster deformation rate, with a sufficiently high viscosity at a low deformation rate (Chen et al., 2022).
Besides the formulation, temperature is a convenient tool to control the ink rheology (Chen et al., 2022). For example, chocolates heated above the melting temperature can flow and therefore be printed. Further, solidification after storing the printed product at ambient or lower temperatures enables network formation (high viscosity or yield stress) and thus the designed shape.
In addition, texture plays an important role in sensorial and structural properties of the printed products, as a hardness value ranging from 4.48 to 59.8 Newtons and a springiness value under 1 have been reported for many successful prints (Zhang et al., 2021). Physical and chemical phenomena of the constituent ingredients during storage and after thermal treatments are also expected to change sensorial and quality properties of the printed products.
FSTA contains a wealth of reliable, interdisciplinary, food-focused information, making it a great tool for researching published science on viscosity and starch digestion. For example, FSTA content that investigates 3D and 4D printing of food products includes over 700 records of high-quality content directly relevant to these additive manufacturing processes across the whole database
Read more facts about FSTA content on this topic, including key journals and FSTA descriptors.
Extension — 4D food printing
The concept of “4D printing” was first proposed by Professor Skylar Tibits at the Massachusetts Institute of Technology in 2013 based on the extension of 3D printing technologies by incorporating a “space-time axis” as the fourth “D” (Tibits, 2014).
Different from non-food sectors utilizing smart materials, e.g. those that can “memorize” their shape before printing and recover their shape after printing, 4D food printing currently utilizes known physical and chemical properties of food materials as a function of stimuli such as moisture content, temperature, pH, and electromagnetic radiations to change the shape, texture, colour, and release properties of entrapped flavourings, nutrients, and bioactive compounds (Teng et al., 2021).
There are several fascinating examples that, by engineering food chemistry and physics, 4D-printed foods can provide novel ideas for designing interactive foods to enhance the eating experience. For 3D-printed chocolate resembling a cocoa bean, pouring a hot chocolate sauce induces a blooming to enable a personalized experience of enjoying desserts (Figure 4; https://www.dezeen.com/2020/03/21/3d-printing-chocolate-barry-callebaut/).
Figure 4: The blooming of a 3D-printed chocolate cocoa bean after pouring a hot chocolate sauce (Source: Mona Lisa 3D Studio https://www.barry-callebaut.com/en/artisans/mona-lisa/3dstudio)
Another example is the bending of purple sweet potato purees during microwave dehydration with the rate controllable by changing microwave power, salt content or fructose syrup content, leading to personalized shapes (Figure 5) (He et al., 2020a).
Figure 5: Moisture changes (a) and quantitative (b) and visual (c) bending of purple sweet potato purees with different salt content after microwave dehydration for different durations; shape and colour change examples of 3D-printed purees induced by microwave dehydration (d) (He et al., 2020a)
The last example is based on anthocyanins that are pigments in purple sweet potato purees. Anthocyanins change the colour, from red to blue when pH changes from 2.0 to 10.0 (Schwartz et al., 2017). After printing mashed potato prepared with 2% w/v sodium alginate and different amounts of potato flakes (pH 6.5) on purple sweet potato puree, the gradual diffusion of anthocyanins into the mashed potato layers with and without the prior addition of 1% citric acid (pH 2.5) or 1% sodium bicarbonate (pH 7.8) resulted in different colours and intensities over time (Figure 6) (He et al., 2020b).
Figure 6: Time lapse colour changes of a puree layer containing 2% sodium alginate and 15-27% mashed potato without (pH 6.5) or with 1% citric acid (CA, pH 2.5) or 1% sodium bicarbonate (SB, pH 7.8) printed on a layer of purple sweet potato puree. Anthocyanins, purple pigments, diffusing to the top mashed potato layer induces pH-dependent colour changes (He et al., 2020b)
This integration of chemistry, physics, and engineering, therefore, enables numerous opportunities for innovation of 3D and 4D food printing technologies, with the possiblity to extend the technology to even higher dimensional printing (Ghazal et al., 2022).
References
- Baiano, A. (2022). 3D Printed Foods: A Comprehensive Review on Technologies, Nutritional Value, Safety, Consumer Attitude, Regulatory Framework, and Economic and Sustainability Issues. Food Reviews International, 38(5), 986–1016. https://doi.org/10.1080/87559129.2020.1762091.
- Chen, Y.Y., Zhang, M., Sun, Y.N. & Phuhongsung, P. (2022). Improving 3D/4D printing characteristics of natural food gels by novel additives: A review. Food Hydrocolloids, 123, 107160. https://doi.org/10.1016/j.foodhyd.2021.107160.
- Dai, X., Liu, L., Ouyang, J., Li, X., Zhang, X., Lan, Q., & Xu, T. (2017). Coaxial 3D bioprinting of self-assembled multicellular heterogeneous tumor fibers. Scientific Reports, 7(1), 1457. https://doi.org/10.1038/s41598-017-01581-y.
- Dankar, I., Haddarah, A., Omar, F.E.L., Sepulcre, F. & Pujola, M. (2018). 3D printing technology: The new era for food customization and elaboration. Trends in Food Science & Technology, 75, 231–242. https://doi.org/10.1016/j.tifs.2018.03.018.
- Ghazal, A.F., Zhang, M., Mujumdar, A.S. & Ghamry, M. (2022) Progress in 4D/5D/6D printing of foods: applications and R&D opportunities. Critical Reviews in Food Science and Nutrition. https://doi.org/10.1080/10408398.2022.2045896.
- He, C., Zhang, M. & Devahastin, S. (2020a). Investigation on spontaneous shape change of 4D printed starch-based purees from purple sweet potato as induced by microwave dehydration. ACS Applied Materials and Interfaces, 12(34), 37896–37905. https://doi.org/10.1021/acsami.0c10899.
- He, C., Zhang, M. & Guo, C.F. (2020b). 4D printing of mashed potato/purple sweet potato puree with spontaneous color change. Innovative Food Science & Emerging Technologies, 59, 102250. https://doi.org/10.1016/j.ifset.2019.102250.
- Liu, Z.B., Zhang, M., Bhandari, B., & Wang, Y.C. (2017). 3D printing: Printing precision and application in food sector. Trends in Food Science & Technology, 69, 83–94. https://doi.org/10.1016/j.tifs.2017.08.018.
- Pereira, T., Barroso, S. & Gil, M.M. (2021). Food Texture Design by 3D Printing: A Review. Foods, 10(2), 320. https://doi.org/10.3390/foods10020320.
- Schwartz, S.J., Cooperstone, J.L., Cichon, M.J., von Elbe, J.H. & Giusti, M.M. (2017). Colorants. In Fennema’s Food Chemistry, 5th Ed. Damodaran, S. and Parkin, K.L. (Editors). CRC Press, Boca Raton, FL.
- Teng, X., Zhang, M. & Mujumdar, A. S. (2021). 4D printing: Recent advances and proposals in the food sector. Trends in Food Science & Technology, 110, 349–363. https://doi.org/10.1016/j.tifs.2021.01.076.
- Tibbits, S. (2014). 4D printing: Multi‐material shape change. Architectural Design, 84(1), 116–21. https://doi.org/10.1002/ad.1710.
- Zhang, J.Y., Pandya, J.K., McClements, D. J., Lu, J. & Kinchla, A.J. (2021). Advancements in 3D food printing: a comprehensive overview of properties and opportunities. Critical Reviews in Food Science and Nutrition, 2, 1–18. https://doi.org/10.1080/10408398.2021.1878103.
Researching 3D and 4D food printing technologies in FSTA
The volume of published research and patents in the area of 3D and 4D printing of food products has increased markedly over the past 5 years, and this is reflected in the growth of FSTA content in this field.
Over 700 FSTA records of high-quality content directly relevant to these additive manufacturing processes have been added since 2017 and the volume is increasing year on year by ~35%.
FSTA indexes more than 550 research articles and 85 reviews. These are sourced from more than 135 quality-checked journals produced by 58 established publishers in 20 countries. As well as research from key international food journals, such as Journal of Food Engineering (ISSN: 0260-8774), Food Hydrocolloids, (ISSN: 0268-005X) and Innovative Food Science and Emerging Technologies (ISSN: 1466-8564), FSTA also includes content on additive manufacturing from key journals in allied disciplines such as International Journal of Biological Macromolecules (ISSN: 0141-8130), Analytical Methods (ISSN: 1759-9679), Analytical and Bioanalytical Chemistry (ISSN: 1618-2642) and Chemical Engineering Journal (ISSN: 1385-8947).
In addition to the journal content, more than 100 patent documents have been indexed, including US, PCT, European and Japanese documents, along with books, book chapters, reports and theses.
To facilitate the discovery of this research content on additive manufacturing in FSTA, we have recently included new subject headings to tag the FSTA content, including 3D Printing, 3D Printed Foods, 4D Printing and 4D Printed Foods. As with all emerging topics, the IFIS Science Team continue to monitor the field and update the subject headings so that users can retrieve comprehensive and relevant content both efficiently and effectively.